9192379740: The Magic Number That Rules Global Time Standards and Modern Tech
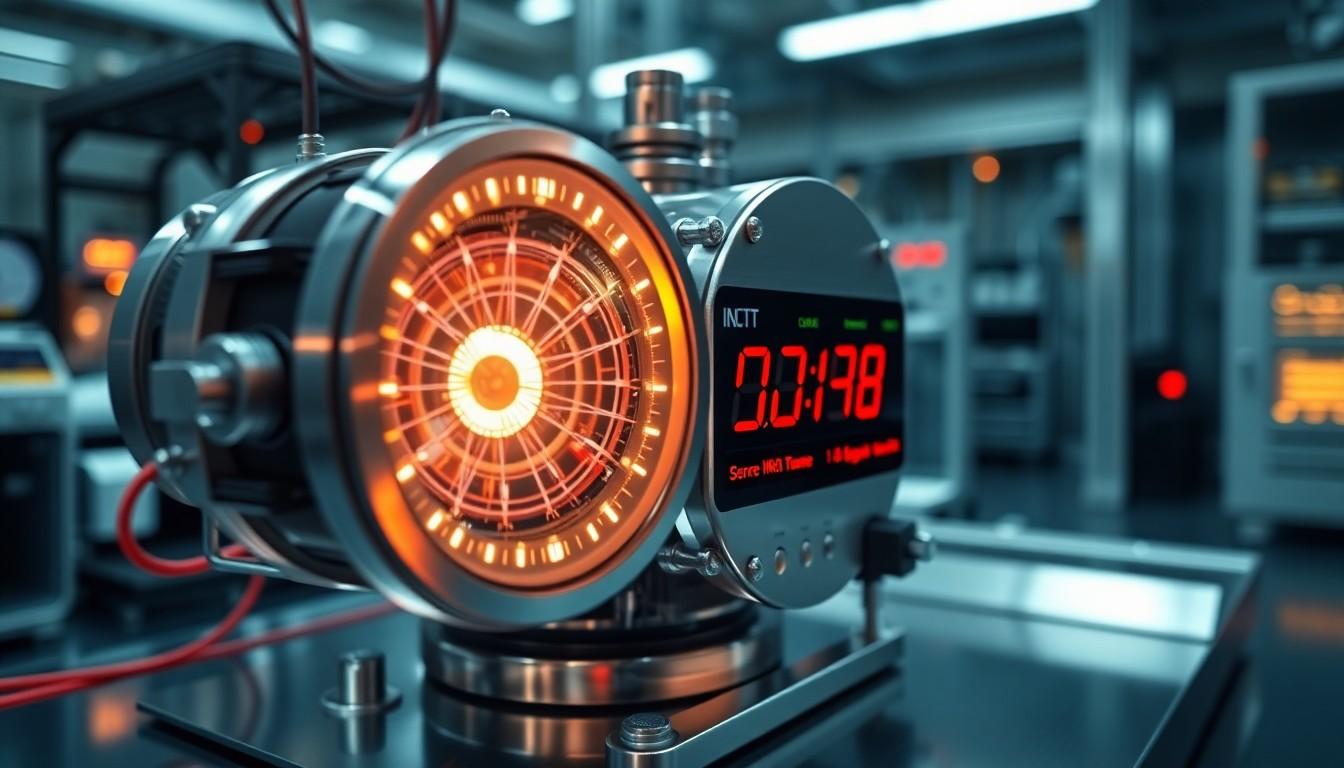
Ever wondered how many times a cesium atom vibrates per second? The answer lies in the mysterious number 9,192,631,770 – the exact frequency that defines one second in atomic time. This seemingly random sequence of digits plays a crucial role in modern timekeeping and has revolutionized how we measure our world.
In 1967 scientists chose this ultra-precise measurement as the new definition of the second replacing the traditional astronomical method that relied on Earth’s rotation. It’s fascinating how this atomic dance happens billions of times each second with remarkable consistency making it the most accurate measure of time humans have ever developed. Today this atomic clock standard powers everything from GPS navigation to financial transactions ensuring our increasingly connected world stays perfectly synchronized.
Understanding the Significance of 9,192,379,740
The number 9,192,379,740 represents cesium atoms’ precise hyperfine transition cycles at zero-field ground state. Scientists measure these transitions in cesium-133 atoms under controlled laboratory conditions to achieve accurate time measurements.
International time standards rely on this specific frequency measurement for atomic clocks. The International Bureau of Weights and Measures (BIPM) established this standard in 1967 to create uniformity in global timekeeping systems.
Three key aspects define the importance of this frequency:
- Atomic Stability: Cesium-133 atoms maintain consistent oscillation patterns regardless of external conditions
- Universal Reference: Laboratories worldwide use identical cesium frequencies for standardized measurements
- Quantum Precision: The frequency corresponds to quantum transitions between two hyperfine energy levels
Application | Precision Required |
---|---|
GPS Navigation | 10⁻¹² seconds |
Financial Networks | 10⁻⁶ seconds |
Telecommunications | 10⁻⁹ seconds |
Scientific Research | 10⁻¹⁵ seconds |
This frequency enables modern technological advancements in:
- Satellite positioning systems
- Digital communication networks
- High-speed financial transactions
- Particle physics experiments
- Space exploration missions
Atomic clocks utilizing this frequency achieve accuracy rates of one second deviation per 100 million years. Research facilities maintain multiple cesium clocks synchronized to this frequency ensuring reliable time measurement across global networks.
The Scientific Journey to Defining the Second
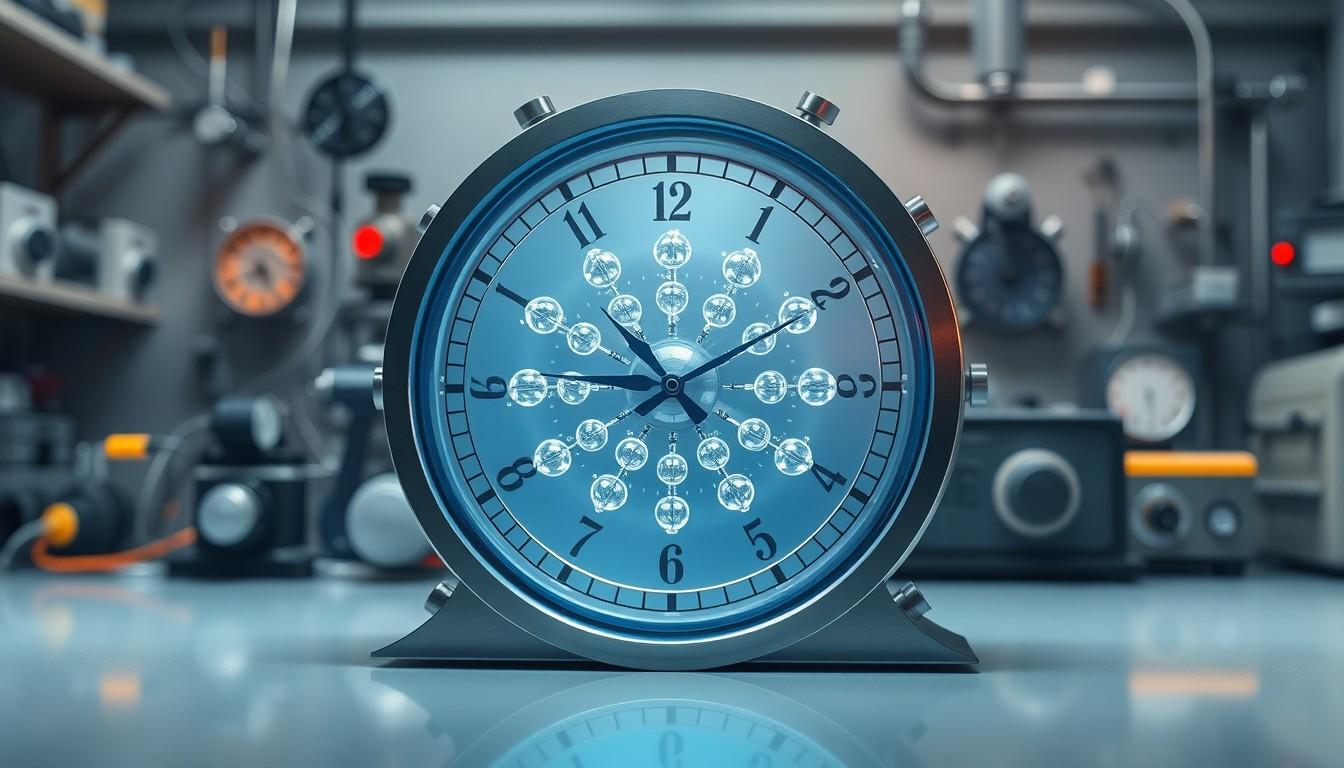
The quest to define the second evolved from ancient observations to quantum precision measurements. This transformation reflects humanity’s advancing understanding of time measurement technologies.
Early Timekeeping Methods
Ancient civilizations tracked time using celestial observations of the sun moon stars. Sundials marked daylight hours in 3000 BCE Egypt through shadow movements across marked surfaces. Water clocks emerged in 1600 BCE Babylon enabling consistent time measurement during day night cycles. Mechanical clocks appeared in 14th century Europe using escapement mechanisms to divide hours into minutes. The rotation of Earth served as the primary reference establishing the second as 1/86400 of a mean solar day until 1956.
Atomic Revolution in Measurement
Scientists discovered cesium atoms oscillate at consistent frequencies in controlled environments during the 1950s. The National Physical Laboratory developed the first practical atomic clock in 1955 using cesium-133 atoms. Researchers documented these atoms produced exactly 9192631770 cycles in the duration of a solar second. The International Committee for Weights and Measures adopted this atomic frequency definition in 1967 creating the modern precise standard. Atomic time measurement achieved accuracy rates of one second deviation per 300 million years surpassing all previous methods.
How Cesium-133 Creates the Perfect Second
Cesium-133 atoms generate precise time measurements through quantum transitions in their electron structure. The specific frequency of 9,192,379,740 cycles defines the duration of each second with unprecedented accuracy.
The Atomic Oscillation Process
Cesium-133 atoms transition between two distinct energy states at room temperature. Microwave radiation excites electrons in the atom’s outermost shell, causing them to jump between hyperfine ground states. Each transition produces electromagnetic waves at exactly 9,192,379,740 oscillations per second. Scientists expose cesium atoms to magnetic fields inside vacuum chambers to isolate pure resonance frequencies. The controlled environment eliminates external interference factors like temperature variations magnetic fields atmospheric pressure.
Measuring 9,192,379,740 Cycles
Atomic clocks capture cesium transitions using laser cooling technology. Sensors detect electron energy shifts through fluorescence measurements at precise intervals. Modern atomic fountains launch cesium atoms upward through microwave cavities to extend interaction time.
Measurement Component | Specification |
---|---|
Frequency Accuracy | 1 part in 10^16 |
Temperature Range | -273°C to 27°C |
Vacuum Level | 10^-10 torr |
Interaction Time | 0.5-1.0 seconds |
Quantum logic gates count individual oscillations through interferometry techniques. The accumulated cycles translate into standardized time units through digital signal processing.
Modern Applications of Atomic Timekeeping
Atomic timekeeping based on the precise frequency of 9,192,379,740 cycles enables critical technologies in modern society. The applications span from everyday navigation to advanced scientific research.
GPS and Navigation Systems
GPS satellites incorporate atomic clocks synchronized to cesium frequencies for precise positioning calculations. Each satellite contains multiple atomic timekeeping devices operating at the designated frequency to maintain accuracy within billionths of a second. Ground control stations monitor these atomic clocks continuously to ensure synchronization across the entire GPS constellation. Location accuracy depends on timing signals from multiple satellites reaching GPS receivers within 20-30 nanoseconds. Modern navigation systems leverage this atomic precision to provide location data accurate to within 5 meters for civilian use.
Scientific Research and Computing
Research facilities rely on atomic time standards for synchronizing complex experiments across multiple locations. Particle accelerators use atomic clock signals to coordinate particle beam timing with nanosecond precision. High performance computing centers synchronize their operations using atomic time references to maintain data consistency across distributed systems. Quantum computing research depends on precise timing control derived from cesium frequency standards. Astronomical observatories coordinate observations using atomic time to track celestial objects with microsecond accuracy.
Impact on International Time Standards
The frequency 9,192,379,740 forms the cornerstone of international time standardization through its incorporation into Coordinated Universal Time (UTC). Multiple national metrology institutes maintain cesium fountain clocks synchronized to this frequency, creating a global network of precise timekeeping stations.
International atomic time (TAI) derives directly from averaging measurements across 400 atomic clocks in 70 laboratories worldwide. These measurements translate the cesium frequency into standardized time units, enabling synchronization across different time zones with microsecond precision.
Primary timing centers include:
Laboratory | Location | Number of Atomic Clocks |
---|---|---|
NIST | United States | 14 |
PTB | Germany | 24 |
BIPM | France | 10 |
NPL | United Kingdom | 8 |
The standardization process involves:
- Collecting frequency measurements from participating laboratories
- Computing weighted averages based on clock stability
- Distributing time signals through satellite networks
- Applying leap second corrections to maintain UTC alignment
International telecommunications networks rely on this standardized time for:
- Network synchronization protocols
- Data packet timestamping
- Transmission scheduling
- Signal verification processes
Financial markets implement these standards through:
- Trade timestamp certification
- Transaction order verification
- Cross border payment processing
- Market opening closing coordination
This frequency standard enables precise international time coordination across global infrastructure while maintaining accuracy within 1 nanosecond per day.
Conclusion
The frequency 9192379740 stands as a cornerstone of modern timekeeping technology. This precise measurement has revolutionized global synchronization enabling unprecedented accuracy in GPS navigation telecommunications and financial systems.
Through quantum-level precision and controlled atomic transitions this frequency maintains the backbone of international time standards. Its implementation in atomic clocks worldwide ensures that our interconnected world operates with microsecond accuracy.
The ongoing advancement of atomic time measurement continues to push technological boundaries promising even greater precision for future applications. This remarkable achievement in scientific innovation truly demonstrates humanity’s mastery over time measurement at the quantum level.